- Eskma Optics 110-0219E, bi-convex
- User Defined Metallic Mirror, concave, 50mm Dia., 100 mm EFL, ideal reflector coating
- Newport 33009FL01-060R, reflective grating, 1200 g/mm
- User Defined Metallic Mirror, concave, 50mm Dia., 100 mm EFL, ideal reflector coating
- Slits: 0.5mm, 1mm, 3mm, and 5mm
- Light Source: Broadband
- Plane Wave
- Wavelength – Top Hat Distribution
- 300-500 nm range
- 10 nm step size
- Power, 1 W
- Unpolarized
- Light Source: Alignment
- Spot: Plane Wave
- Detector: Spectral Analysis
- Spot: Incoherent Irradiance
- Analysis Rays: 1 million
- 200×200 pixels
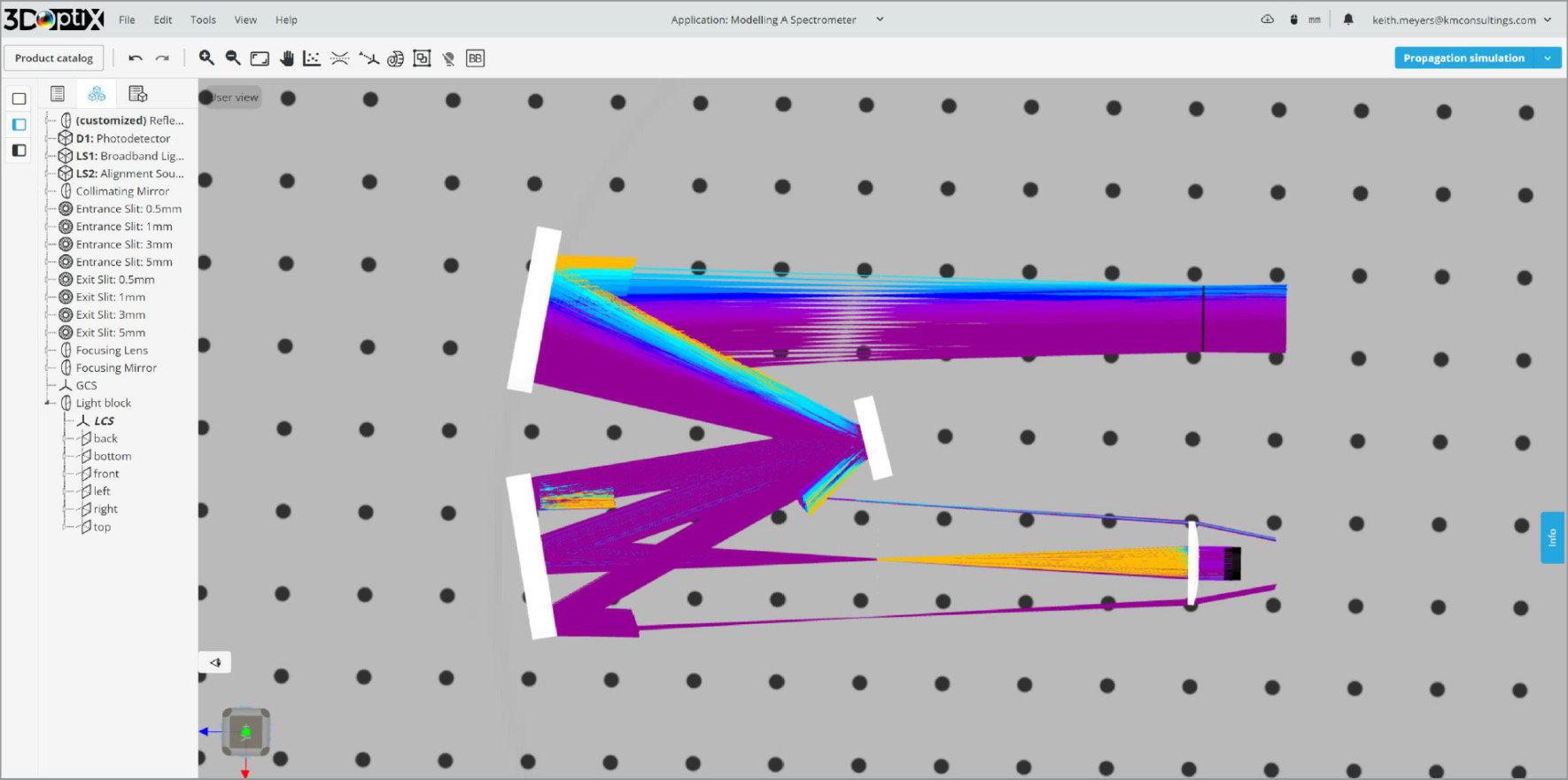
The first step is to create the necessary slits for the system. We will create them in Microsoft PowerPoint for this example, but they can be made in any document program that can specify object dimensions. Of course, we can also make the slits in CAD software and upload them as CAD objects using the IMPORT CAD feature.
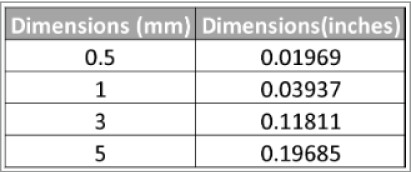
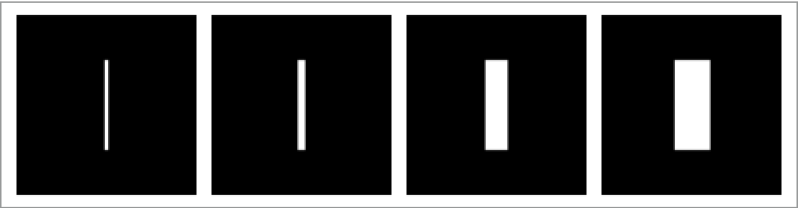
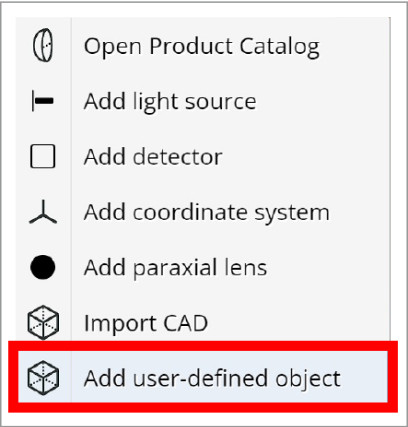
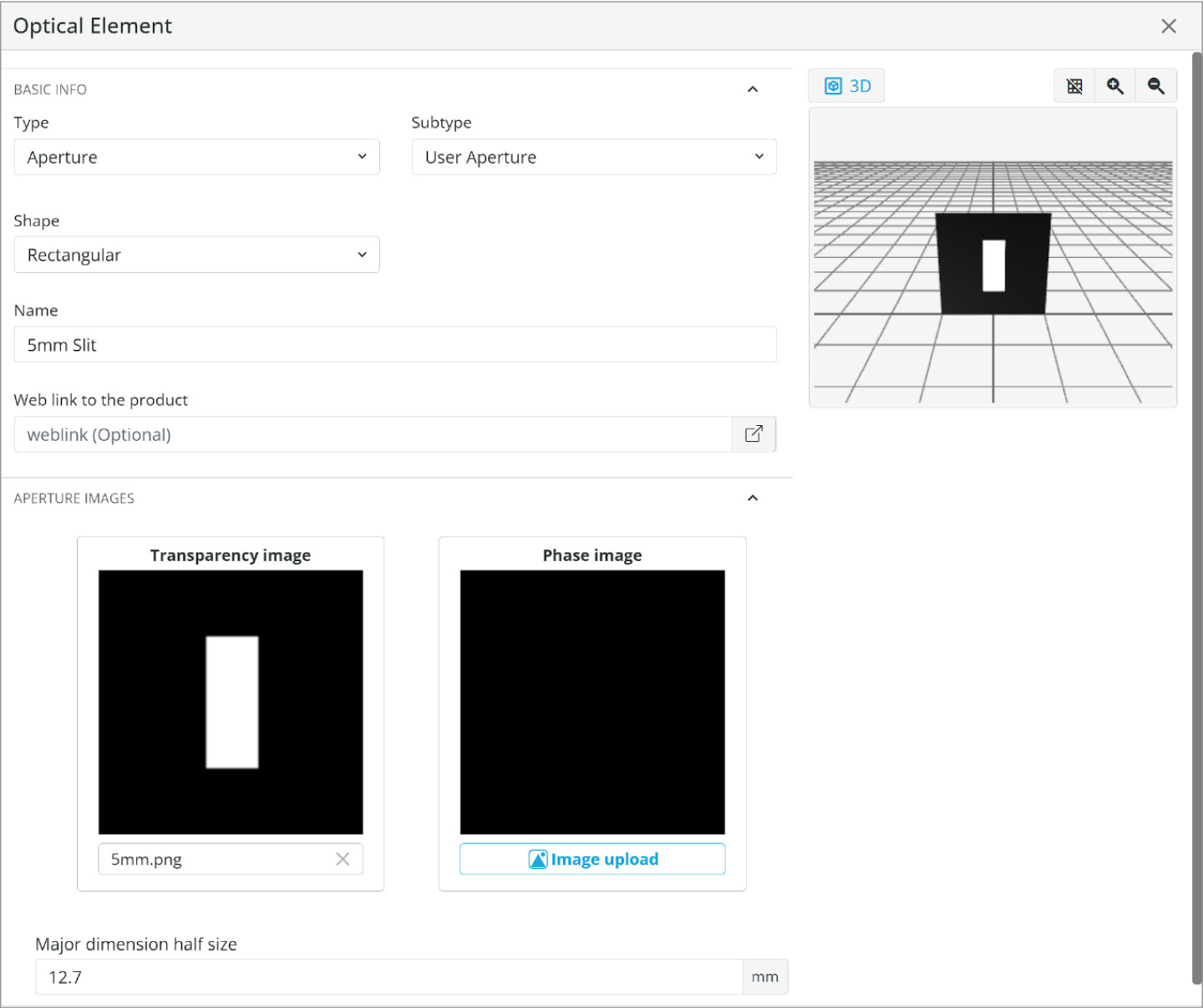
Let’s measure one of the apertures to make sure the import was done correctly. We’ll go to the top bar and select the MEASURE TOOL. We will then click on the center of the slit and both edges.
The measurement tool shows that the aperture was imported successfully with the correct dimensions. This is an important step to ensure that user or import errors do not occur.
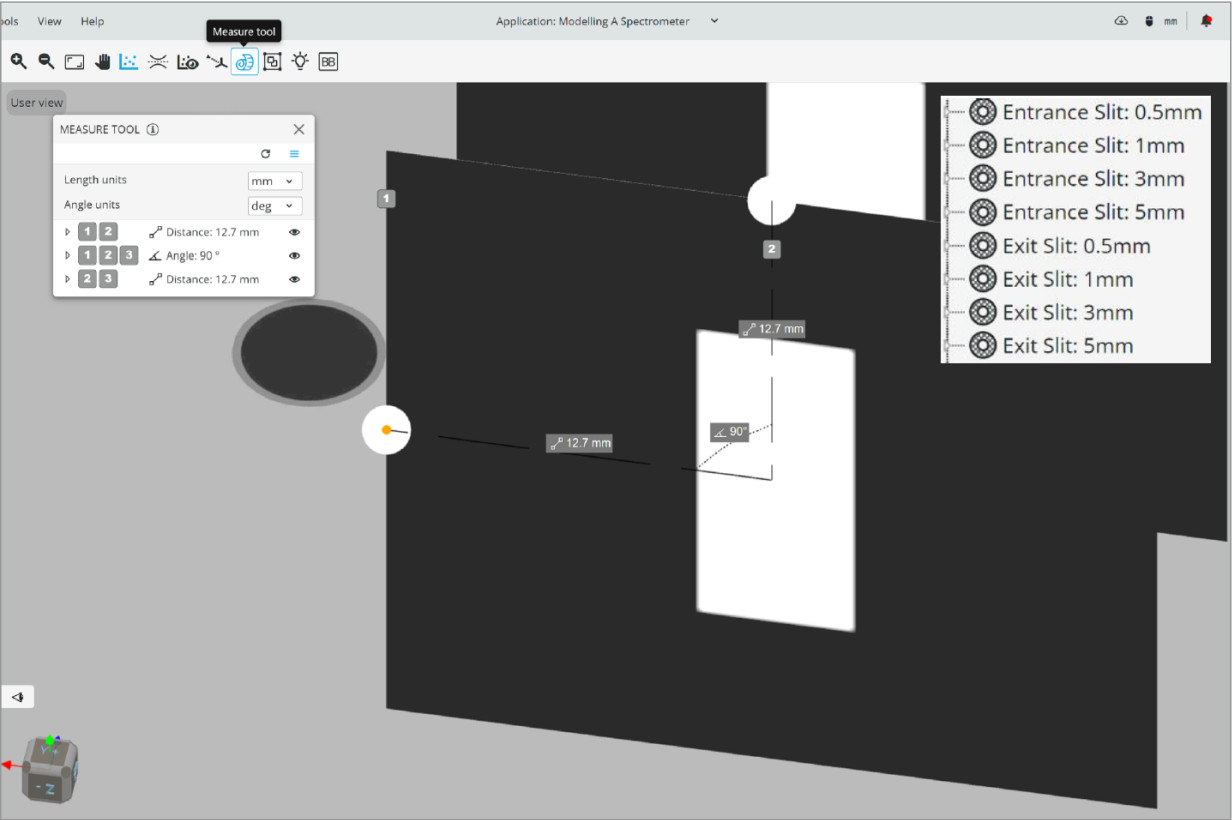
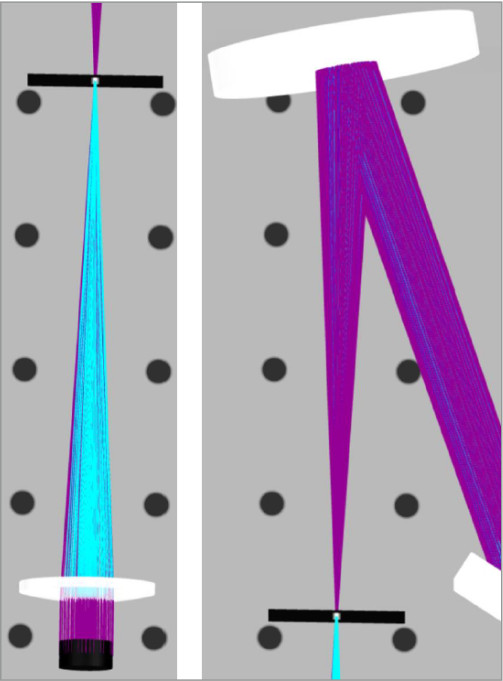
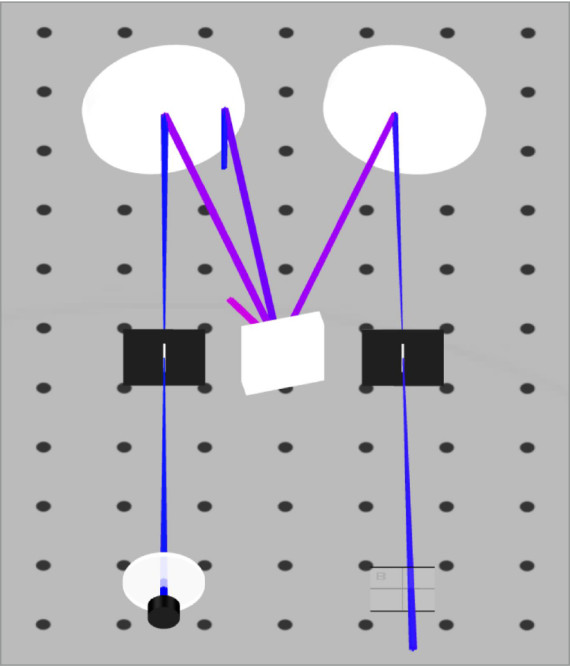
Then the concave collimating mirror on the other side of the slit is also placed at its focal length away from the slit. The mirror is then tilted to direct the light source towards the reflective grating. Since the collimating mirror is a tilted spherical mirror, the optimal position will not be exactly its focal length from the slit. The tilt angle of the mirror is minimized to reduce aberrations.
The grating is placed so that the collimated light from the first mirror is incident on its center, and then tilted to redirect higher diffracted orders of light towards the focusing mirror; mirror 2. The zeroth order diffraction carries all the wavelengths and is not useful to analyze.
The second mirror is then positioned in line with the first mirror horizontally and shifted until the diffracted order of interest is incident on the exit slit. The exit slit is vertically aligned with mirror 2 and horizontally aligned with the entrance slit. The detector is placed horizontally across from the focusing lens and then vertically in line with Mirror 2 and the exit slit.
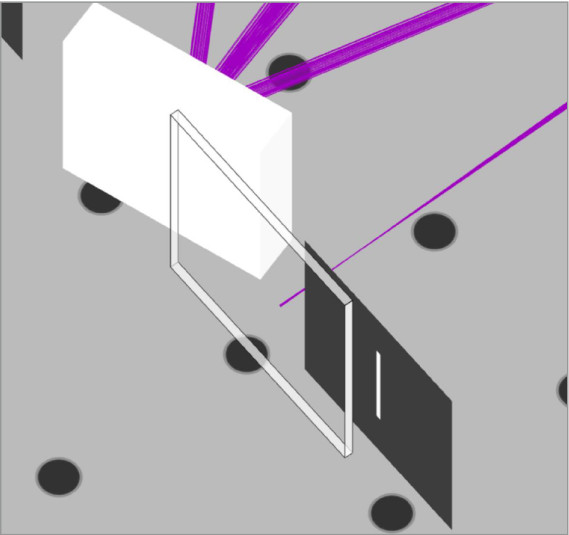
Now that the spectrometer is set up, we can now analyze the system for sensitivity and resolution.
The resolution of a spectrometer is often determined, in part, by the width of the entrance and exit slits. A narrower slit provides better resolution, as it allows less light of various wavelengths to pass through. However, this also means that less light reaches the detector, reducing sensitivity.
Another factor influencing resolution is the groove density of the grating. Higher groove densities can provide better spectral resolution, but they also disperse the light over a broader area, potentially reducing the amount of light collected by the detector and possible overlapping of orders.
One final addition to the optical system will be a light block to fill the gap between the exit aperture and the grating. We will use the ADD USER-DEFINED OBJECT again to place a flat rectangular window with the total absorber coating to the front. This will stop any stray light from reaching the detector.
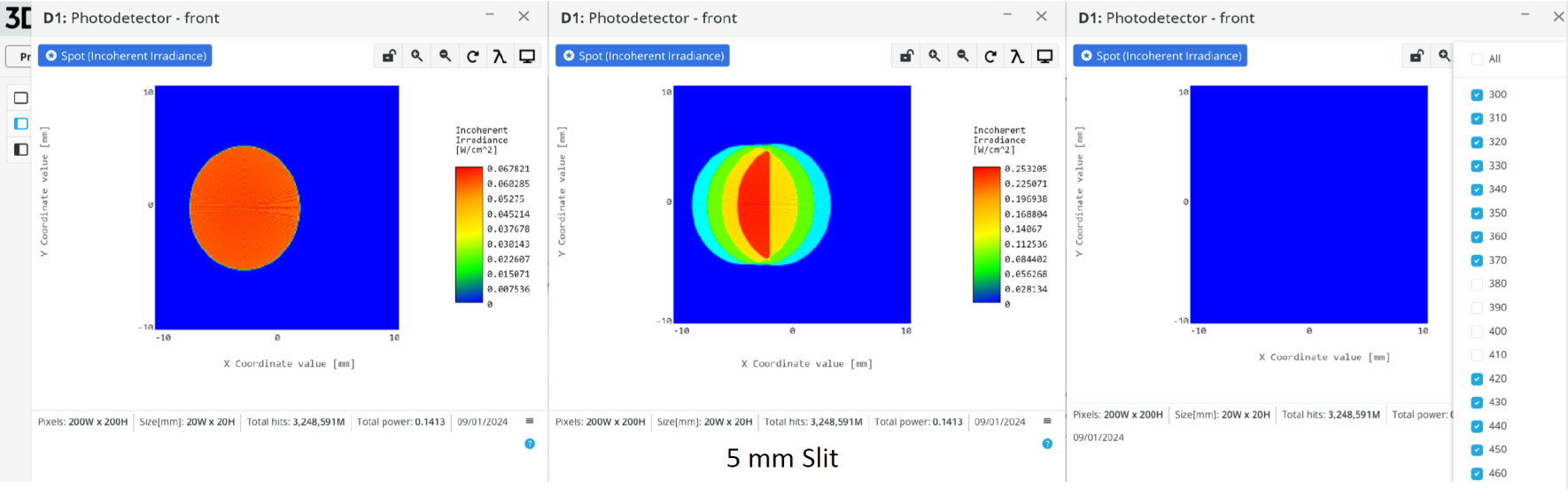
The left detector shows only the center wavelength of 400 nm. This is not obscured by the slit, so the total relative energy that makes it to the detector is 100%, but this includes power from all wavelengths.
The center detector shows all the wavelengths that make it through the slit. As additional wavelengths would be measured by the photodetector this would skew the power measurement to the high side.
The detector on the right shows the wavelengths that do not reach the detector and are completely obscured by the slit. Ideally, the slit we want to choose obscures all wavelengths except the one to measure.
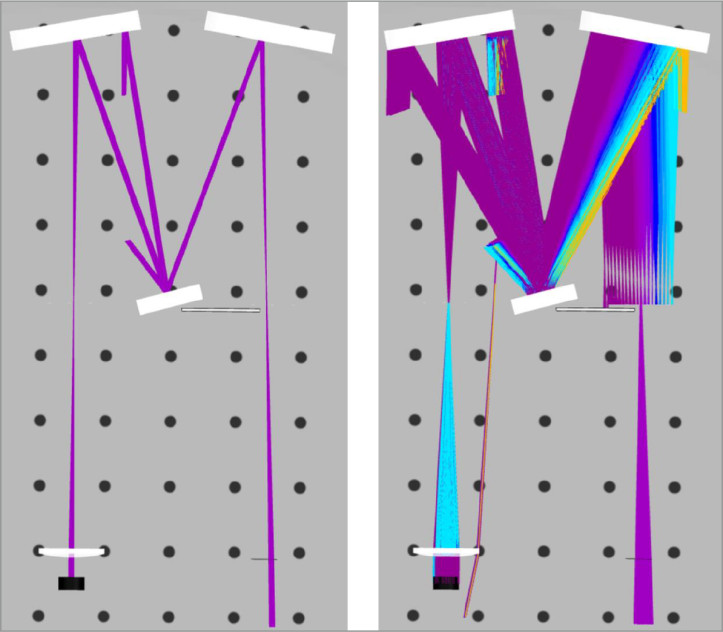
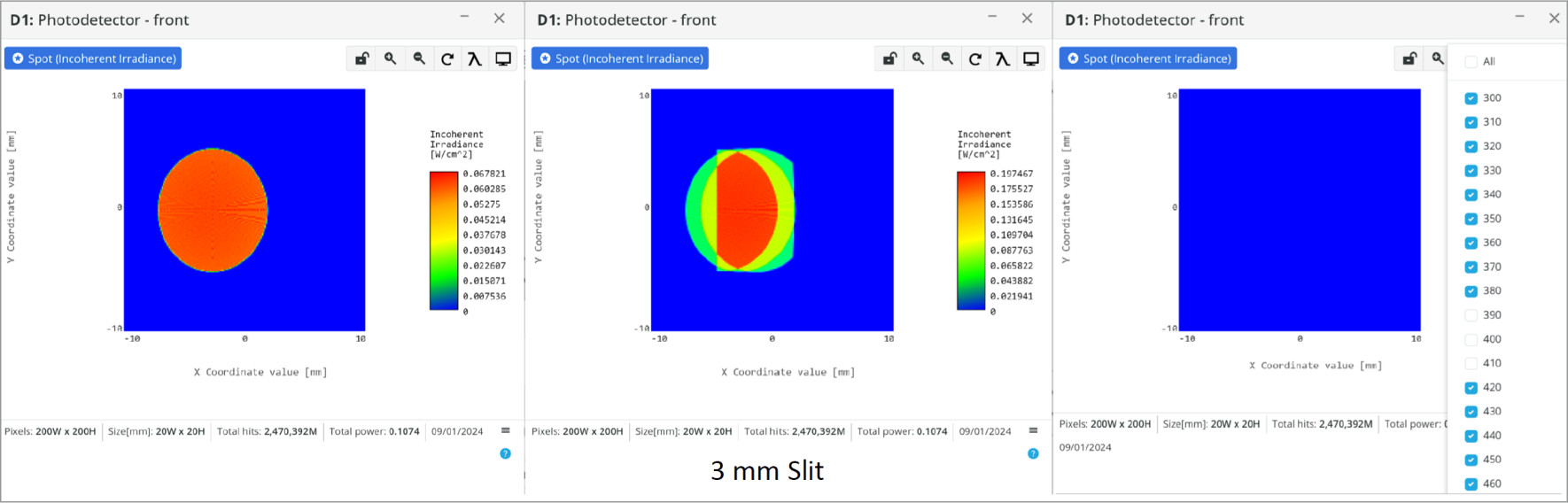
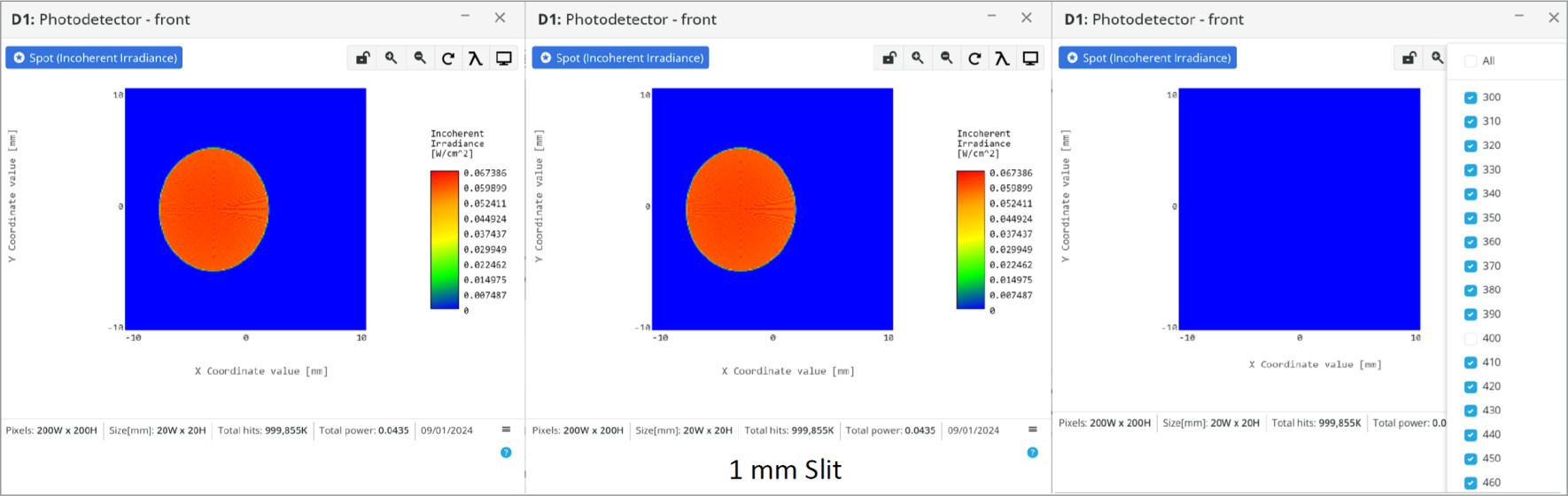
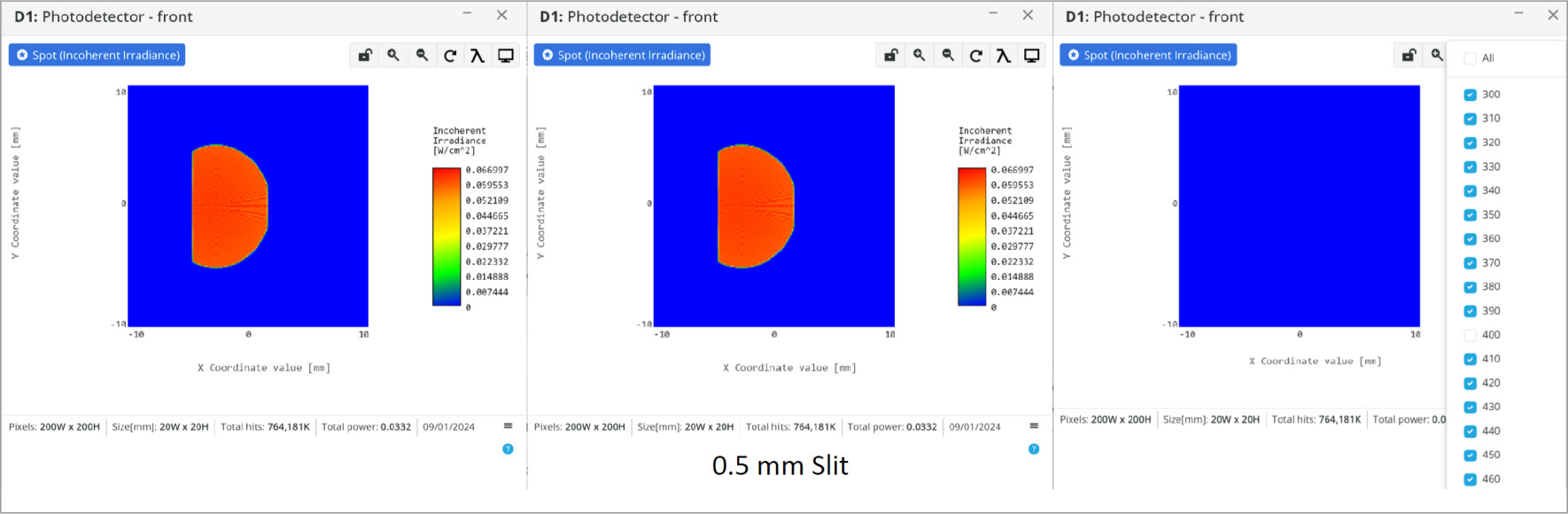
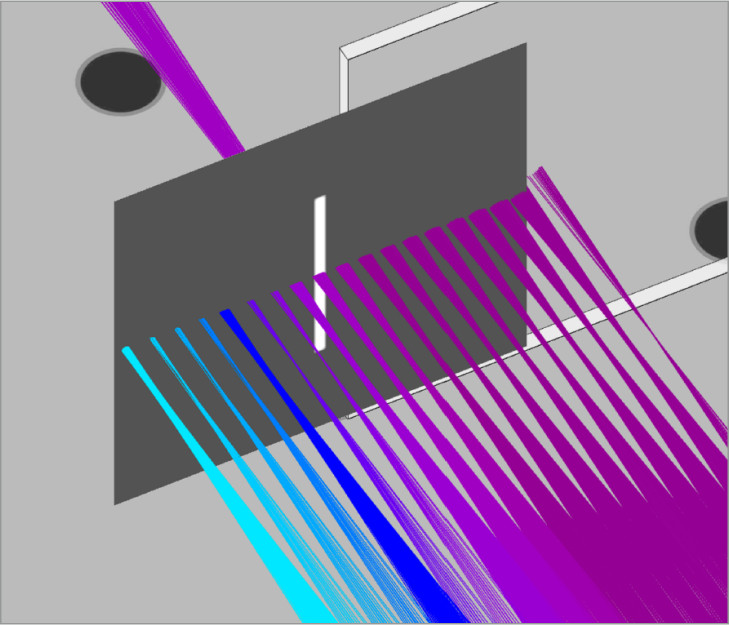